Airfoil Design
- Krish Pesswani
- Jan 8
- 3 min read
Updated: Apr 5
Today on AeroIntellect, we are going to be talking about the design of an airfoil shape and its impact on lift.
To begin with, what is an Airfoil shape? An Airfoil shape is the cross-sectional shape of an aircraft wing. It is primarily responsible for generating lift force, which acts perpendicular to the direction of the airflow; how, you may ask? The answer lies in Bernoulli's principle, which states that as the speed of a fluid increases, its pressure decreases. So if the speed of airflow on top of the wing increases, pressure decreases, thereby producing lift. With that little piece of knowledge, let's move on. Airfoils come in a variety of shapes, each optimized for specific flight conditions and performance requirements. Today, we will discuss the two most common ones: cambered and symmetrical airfoils. Before that, it is essential to know the key features of an Airfoil.
Key Features of an Airfoil
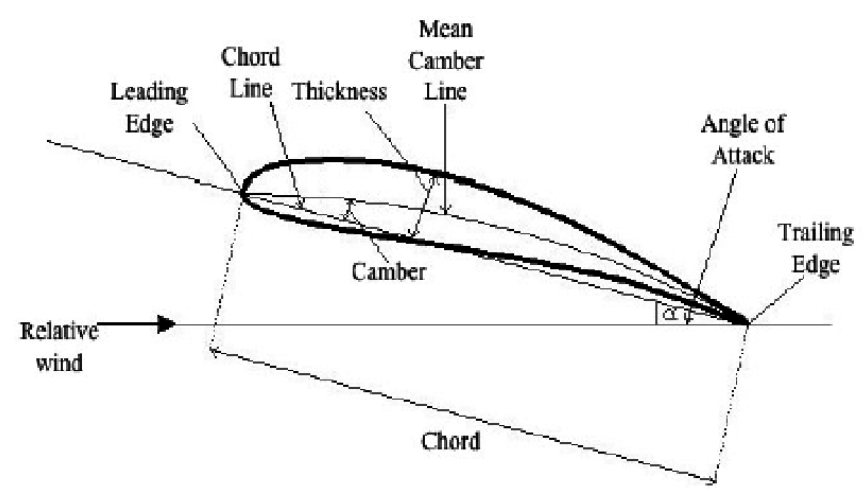
Leading Edge: The front-most part of the airfoil where airflow first makes contact.
Trailing Edge: The rear-most part of the airfoil where airflow exits.
Chord Line: An imaginary straight line connecting the leading and trailing edges.
Chord Length: The distance between the leading and trailing edges along this line.
Camber: The curvature of the airfoil's upper and lower surfaces.
Thickness: The maximum distance between the upper and lower surfaces, usually expressed as a percentage of the chord length.
The angle of Attack (AoA): The angle between the chord line and the relative airflow direction.
Symmetrical Airfoils
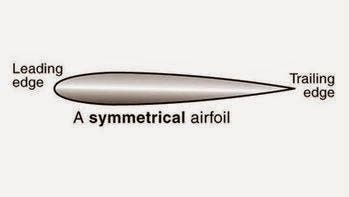
A symmetrical airfoil has the same curvature on the upper and lower surface, as shown in the following image. Now, you might be wondering how lift can be engendered when there is no difference in airflow speed above and below the wing due to a perfectly symmetrical airfoil. The simple answer is that it can't; a symmetric airfoil produces no lift at zero angle of attack (AoA) because the air travels symmetrically over the upper and lower surfaces.
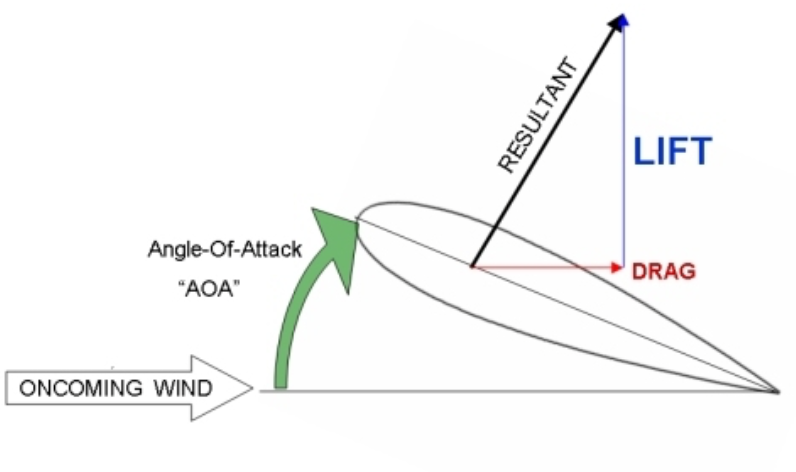
At a positive AoA, air accelerates over the upper surface, creating lower pressure according to Bernoulli's principle. The pressure on the lower surfaces remains relatively higher, resulting in an upward net force known as lift. Symmetrical Airfoils also deflect airflow downward as it passes, creating an equal and opposite upward reaction (Newton's third law). This flow deflection contributes significantly to the lift being caused. Compared to cambered airfoils, symmetric airfoils produce less drag, making them ideal for high-speed aircraft.
However, symmetrical airfoils aren't used in typical passenger airlines; they are widely used in helicopter rotor blades. Now, these blades aren't pre-set to an angle of attack; instead, they are dynamically adjustable during flight using the control systems to meet the diverse aerodynamic demands of flight.
Cambered Airfoils
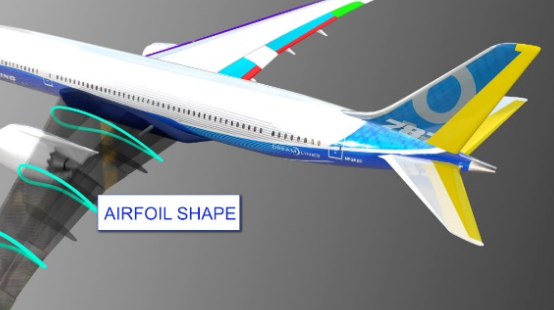
A cambered airfoil has a more curved upper surface than the lower surface. The main difference between symmetrical and cambered airfoil is that cambered airfoil produces lift even at zero or low angles of attack. These are widely used in passenger airlines, such as Boeing 737 and Airbus A320.
You are probably curious: if the wings are pre-built in a way that doesn't require any AoA to induce lift, why doesn't it take off before or after the actual takeoff time? Well, AoA still plays a critical role, alongside takeoff speed, environmental conditions, etc. This is because the airflow over the wing is insufficient to create enough lift to counteract the aircraft's weight at a lower speed. So, if you are asking yourself, what changes in the aircraft when the pilots pull back on the control stick? The AoA is being increased to overcome the weight acting on the plane. Cambered airfoils are optimized to produce efficient lift during cruising at relatively low AoA and high speeds.
All in all, pilots increase the AoA during takeoff because a cambered airfoil alone, at its neutral position, cannot form enough lift at takeoff speeds. Adjusting the AoA ensures the wing produces sufficient lift to overcome the aircraft's weight, allowing it to transition from the ground to flight efficiently.
Ultimately, Bernoulli's principle states that as the speed of a fluid increases, its pressure decreases. When air flows over a wing, the cambered airfoil shape causes the air on top to move faster than the air below. This difference in air creates a pressure difference, where lower pressure exists on top of the wing and higher pressure below. This generates lift.
Thank you for joining us on this exploration of airfoil design. Stay tuned for next week's deep dive into another captivating topic in aerospace engineering!
Very Very nice